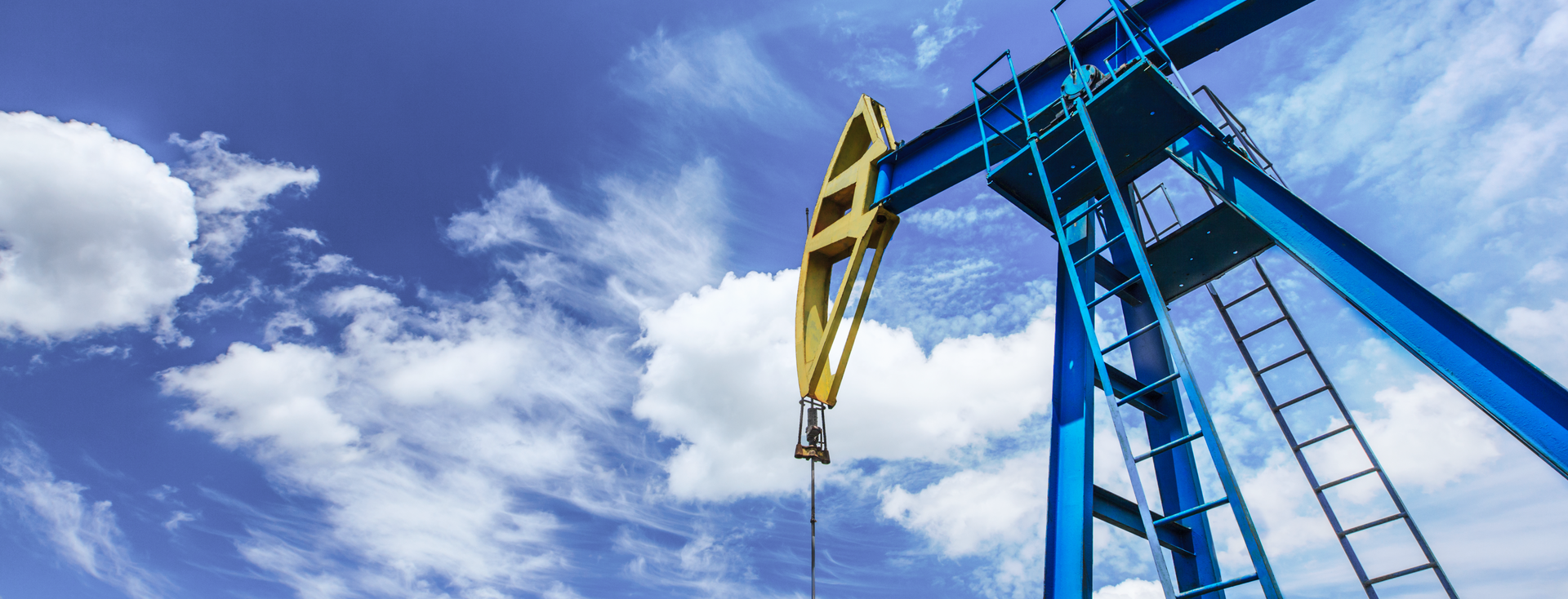
Development of customized demulsifiers
Interfacial rheological measurements on crude oil emulsions
The process of tertiary oil recovery leads to the formation of water-in-oil (w/o) emulsions. Demulsifiers split these emulsions, thus enabling dewatering and desalination of the crude oil. Dewatering is necessary in order to save energy required for processing crude oil, to avoid corrosion problems during preparation for the refinery, and to reduce pressure variations when transporting oil through pipelines.
Interfacial rheological measurements using the oscillating drop method were carried out in order to select suitable demulsifiers for use in practice. This method predicts the structural mechanical stability and therefore the decay of the w/o emulsion when using demulsifiers. The optimized demulsifier was tested in practice in crude oil drilling fields and led to a lower salinity of the oil and a reduction in pump pressure.
Background
The oil production process leads to the formation of water-in-oil (w/o) emulsions. These have essentially two major disadvantages in the subsequent oil recovery process.
1) (w/o) emulsions are more viscous than the oil itself and their transportation (e.g. from the production site to the refinery) demands high pump powers which increase the energy requirement and adversely affect pump life.
2) The dispersed phase of the (w/o) emulsions is chloride-containing brine which causes corrosion problems during preparation for the refinery.
Asphaltenes present in crude oil make a significant contribution to stabilizing such emulsions. Asphaltenes stabilize w/o emulsions as they form a “hard” molecular mesh at the oil-water phase boundary. Such interface films have a visco-elastic character and have a high storage modulus (= modulus of elasticity). This study therefore focuses on the effect of asphaltenes on film stability.
Demulsifiers are used in order to avoid problems which occur due to the formation of (w/o) emulsions in crude oil production. These split the emulsions and make dewatering and desalination of the crude oil possible [1].
The choice of a suitable demulsifier system must be adapted to suit the globally varying composition of crude oil and to whether onshore or offshore drilling is involved. As a consequence of this, extensive optimization of the demulsifier system is necessary which, depending on the price of oil, often makes the use of demulsifiers uneconomic.
To achieve individual matching, demulsifiers are initially analyzed in the laboratory using small sample quantities. If this is satisfactory, it is sufficient to check the result just once based on more extensive investigations in a real environment, i.e. at the oilfield. Exclusive optimization of demulsifiers in the reservoir would be considerably more time-consuming and cost-intensive. In this case, interfacial rheology is a suitable method for investigating and optimizing demulsifier systems in model experiments.
The following explains the methodology of interfacial rheology and shows how to arrive at the optimal results in real crude oilfields from the model experiment. The demulsifier (OU-1 + CAPB) optimized in the model experiment is compared with an established industrial demulsifier (Pralt-11A). Here, it is shown that the optimized demulsifier leads to improved dewatering and desalination and to a reduction in pump pressures compared with Pralt-11A.
Experimental part
Interfacial rheological measurements using the oscillating drop method
Measurement of the dynamic interfacial tension while the drop is simultaneously oscillated forms the basis for determining dilative rheological properties of the interface [2, 3]. Here, the volume of a pendant drop is varied periodically and the resulting interfacial tension calculated as a function of time based on the optically detected drop profile. The characteristic of the interfacial tension in response to the drop oscillation is to a great extent sensitive to demulsifiers at the liquid boundary. For this reason, investigation of the drop oscillation enables a judgment to be made regarding the thickness and strength of an interfacial layer. The measuring principle is shown in Fig. 1.
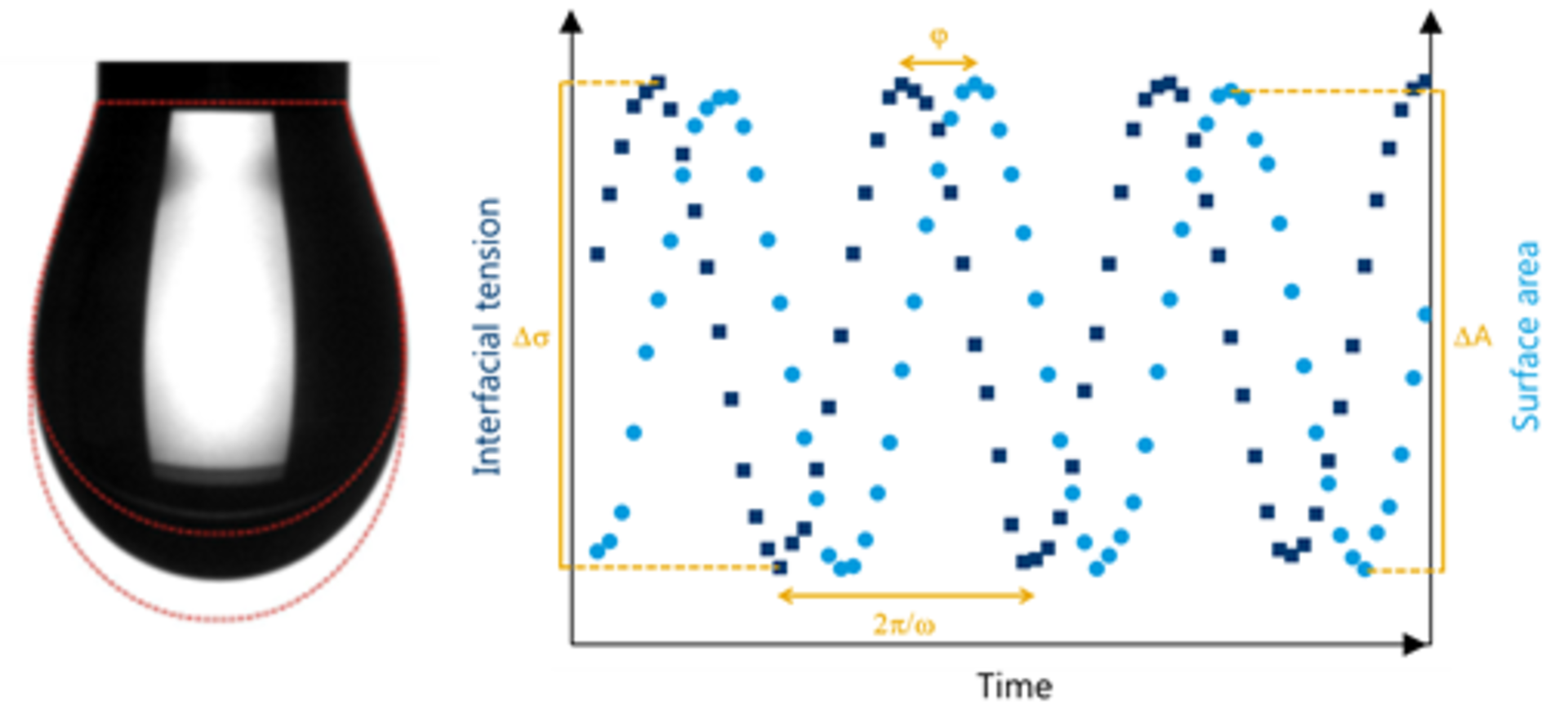
The complex visco-elastic modulus E* can be calculated from the change in drop surface area (ΔA), the mean drop surface area (A0) and the change in interfacial tension (Δσ):
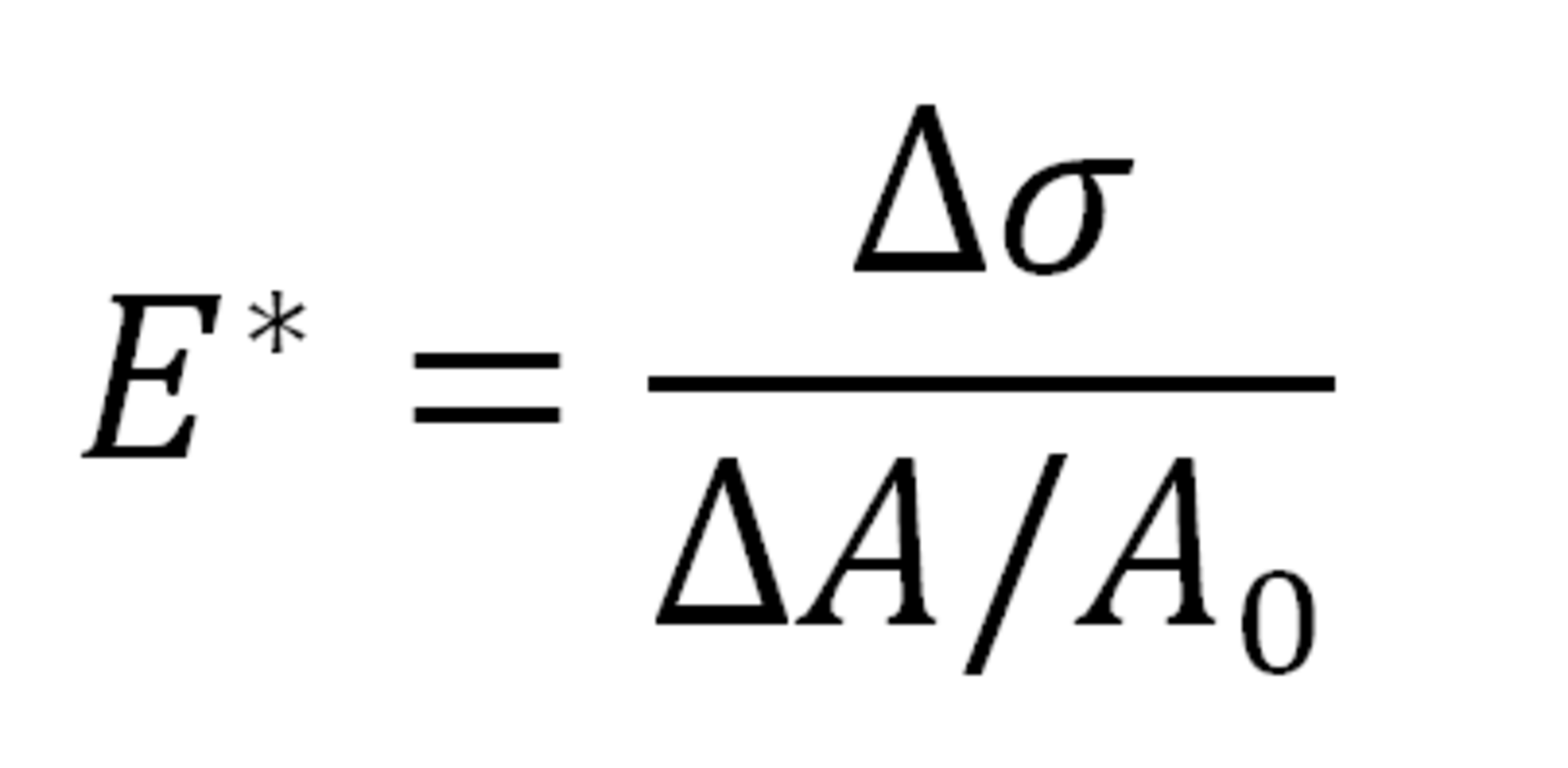
The visco-elastic modulus can be divided into the storage modulus E’ and loss modulus E” by means of the determined phase shift φ:
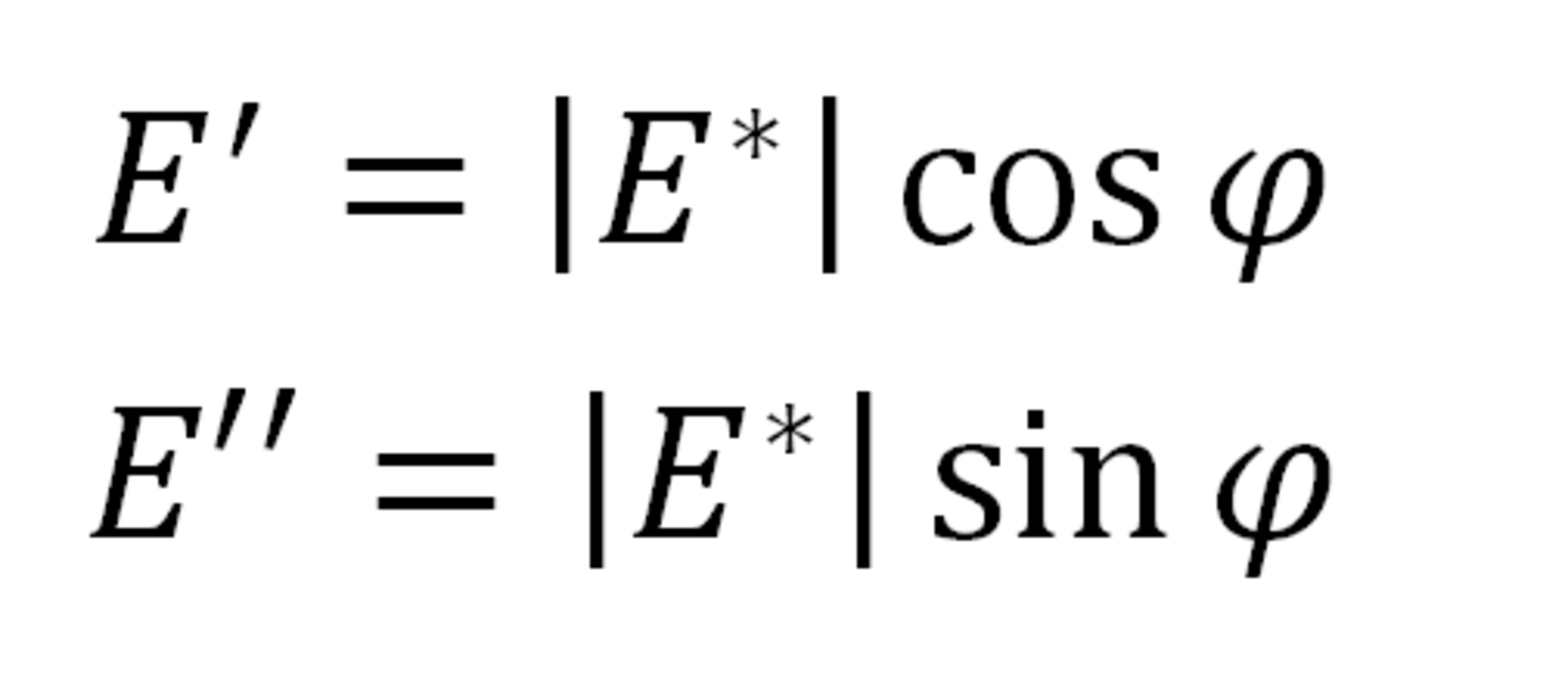
Instrumentation and samples
The rheological properties of different interfaces were investigated with the Drop Shape Analyzer – DSA30 incorporating the additional interfacial rheology module (Oscillating Drop Module, ODM). Toluene solutions with different concentrations of asphaltenes extracted from crude oil were used as the model oil phase. [1]. Drops of the model oil phase were formed in the bulk aqueous phase. Various demulsifiers were also added to the aqueous phase at a later stage. The demulsifier substances investigated are given in Table 1. A sinusoidal oscillation with a period of 5 seconds was used for the drop oscillation. During the oscillation, the variation in drop surface area was ΔAmax / A0 = 10 – 15% [4].
Abbreviation | Name of substance |
OAPB | Oleyl amido propyl betaine |
CAPB | Cocamidopropyl betaine |
AB | Alkyl betaine |
OAPDAO | Oleyl amido propyl dodecyl dimethylamine oxide |
OAPTAC | Oleyl amido propyl trimethyl ammonium chloride |
CTAC | Cetyltrimethylammonium chloride |
OU-1 | Oligourethane |
Tab. 1: Abbreviations of the substances used and their full name.
Results
Rheological properties of interface films
All experiments and results shown below were carried out, obtained and published as part of the doctorate thesis by Mingazov (Kazan National Research Technical University, Russia) [1].
In a first step, the conditions for the formation of stable adsorption films at the interface between the asphaltenes dissolved in toluene and water were investigated with the help of interfacial rheological measurements. In doing so, two parameters were of interest: the asphaltene concentration in the solution and the time to form the said adsorption films.
The change in interfacial tension with respect to time of asphaltene-toluene solutions and water for different asphaltene concentrations was investigated using the pendant drop method (Fig. 2).
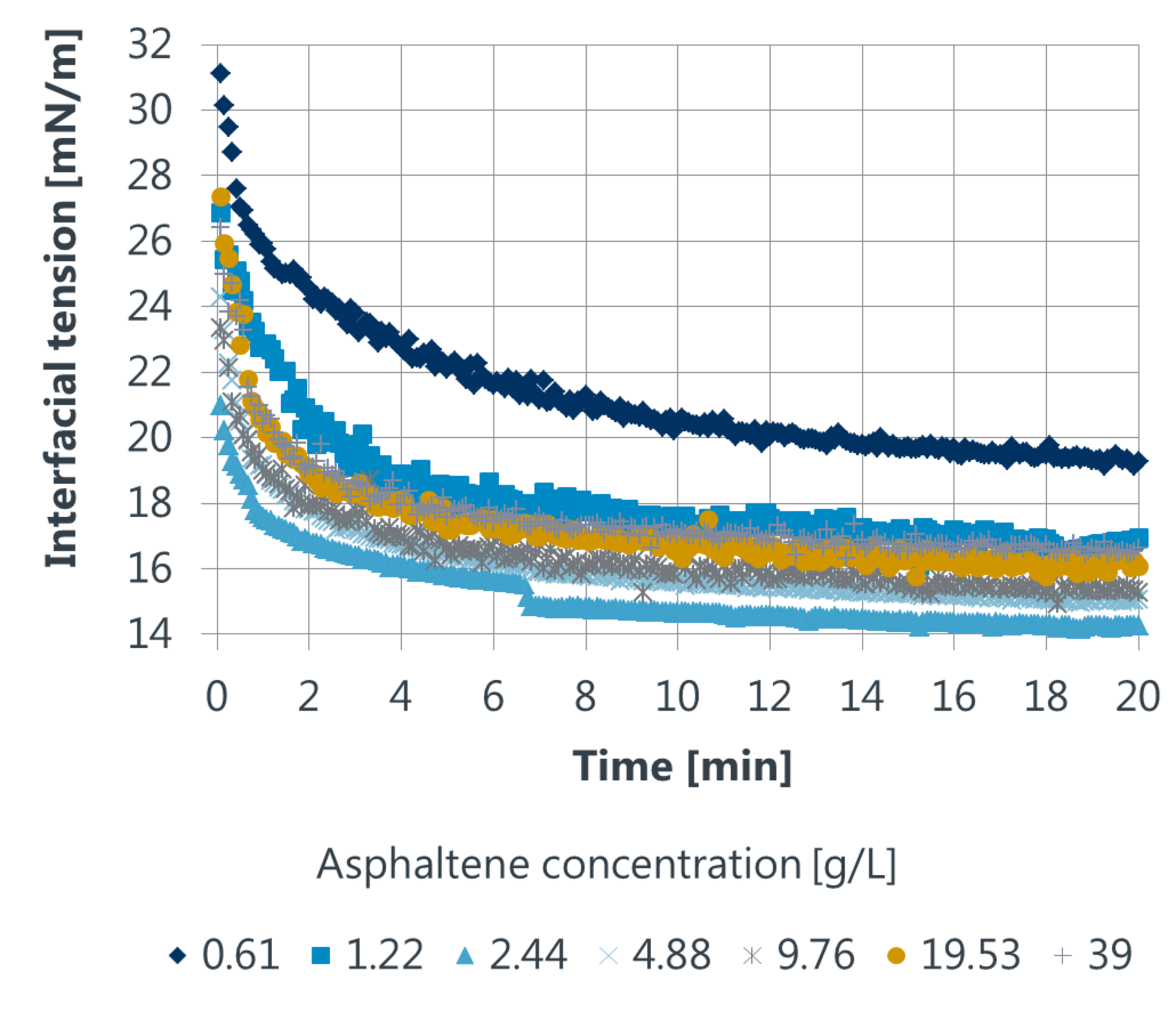
For all concentrations, the interfacial tension decreases with time and after 15 minutes all curves tend to a constant equilibrium value. From the determined equilibrium values, an isotherm for the interfacial tensions was plotted against different asphaltene concentrations (Fig. 3). The isotherm obtained shows a minimum at c = 2.44 g/L, which, in a similar way to critical micelle concentration (CMC), can be associated with the aggregation of asphaltenes in solution.
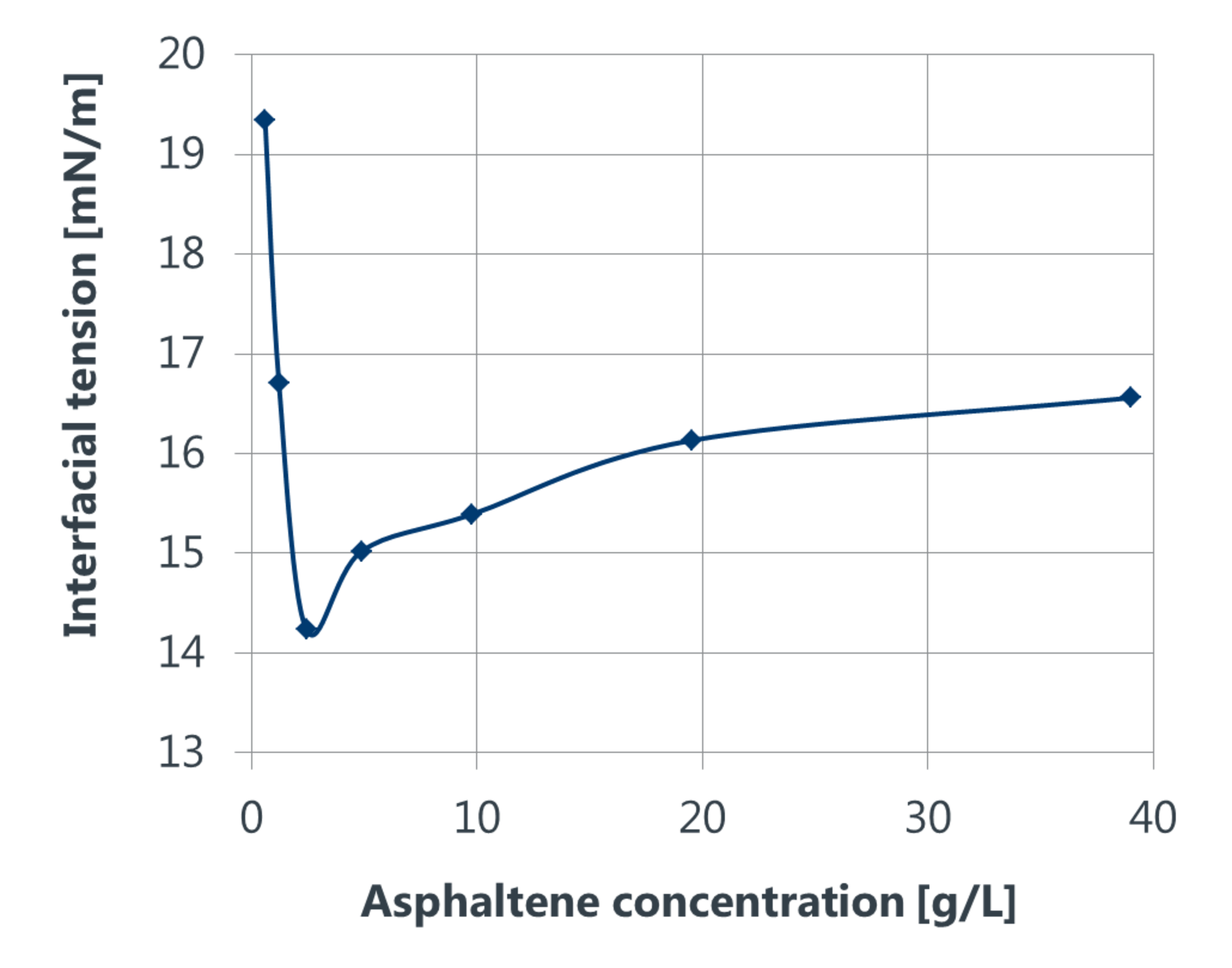
The storage modulus of the interfacial film for an asphaltene concentration has a maximum in a similar way to CMC [4). As changes in the interfacial rheology due to adding demulsifier substances can be shown to be most sensitive at this concentration, further investigations on asphaltene solutions were carried out with concentrations corresponding to the CMC.
Fig. 4 shows the variation of the storage modulus with respect to time for the different demulsifier substances investigated.
An adsorption layer consisting of asphaltenes forms within 20 minutes (initial increase in storage modulus). In the further course of the investigation (up to 100 min), structuring of the asphaltene adsorption layer occurs until the storage modulus has stabilized. After 100 min, different demulsifier substances were added to the aqueous phase, and the variation of the storage modulus with respect to time was investigated. Fig. 4A shows the characteristic for ionic demulsifiers and Fig. 4B that for oligourethane-based mixed systems.
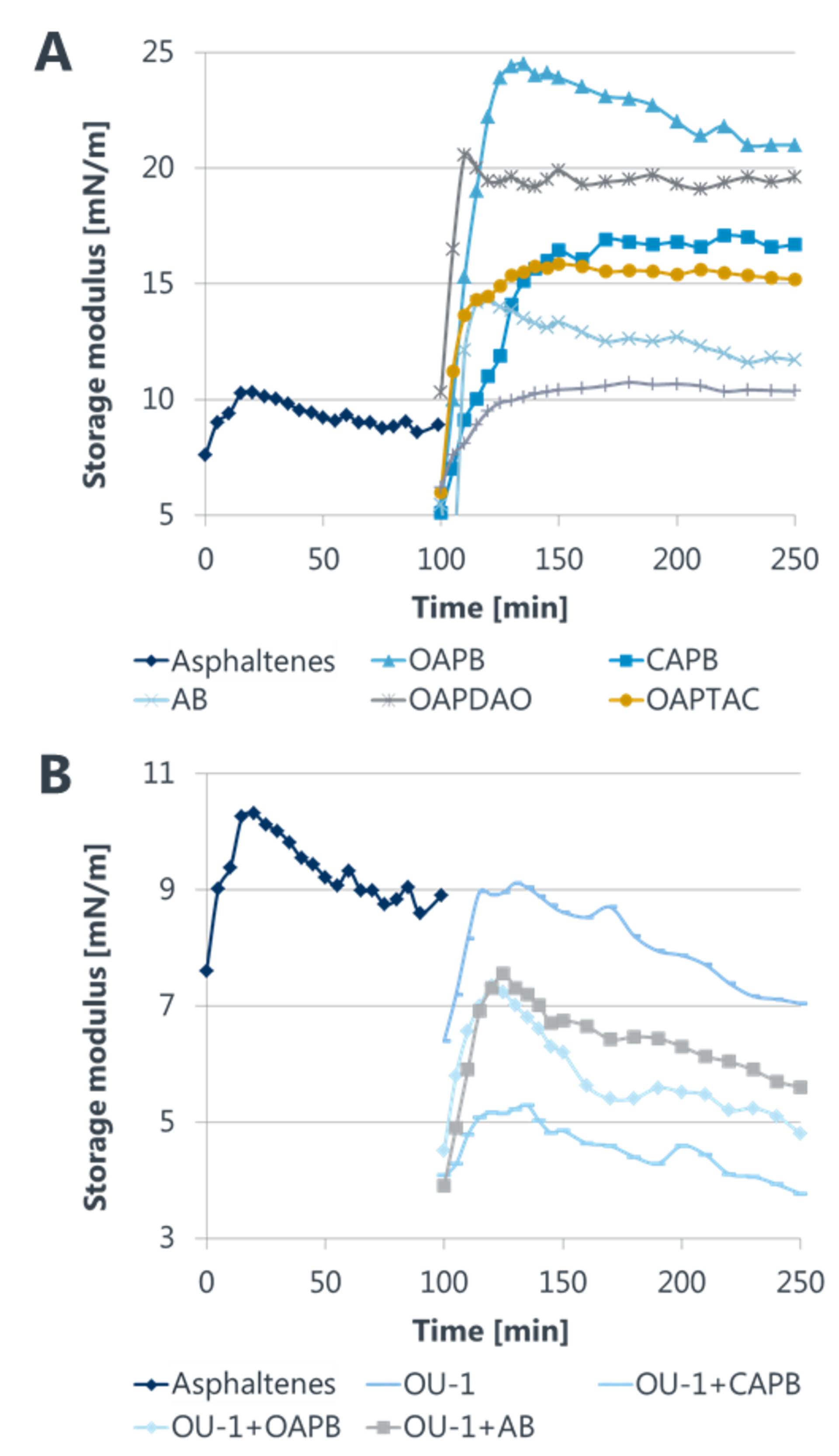
The introduction of ionic surfactants into the model system leads to an increase in the storage modulus (Fig. 4A). Here, OAPB leads to the greatest measured increase. Ionic surfactants are therefore not suitable as demulsifiers for the system under investigation. In contrast to this, the oligourethane-based mixed systems cause a reduction in the storage modulus (Fig. 4B) and are highly suitable as demulsifiers. The mixed system OU‑1 + CAPB shows the lowest value for the storage modulus. This correlates with the lowest structural mechanical strength and leads to the decay of the (w/o) emulsion.
The system OU-1 + CAPB therefore represents the optimum demulsifier from the series of preliminary investigations.
Comparison of two demulsifiers in field trials
The mixture of oligourethanes and ionic demulsifiers (OU-1 + CAPB) optimized as a result of preliminary experiments therefore leads to a reduction in the structural mechanical strength of the emulsions. This optimized demulsifier system was subsequently tested in a field trial.
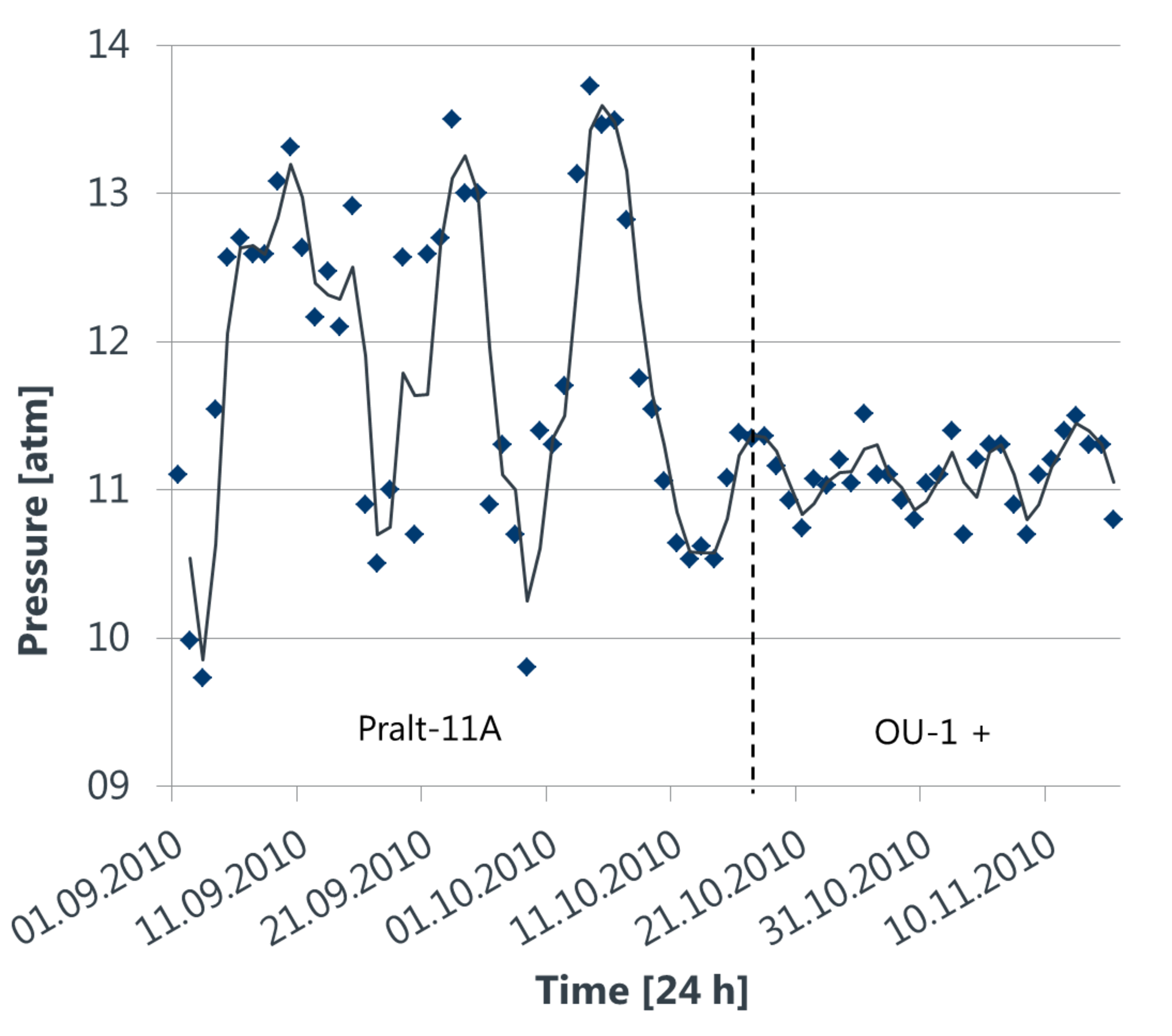
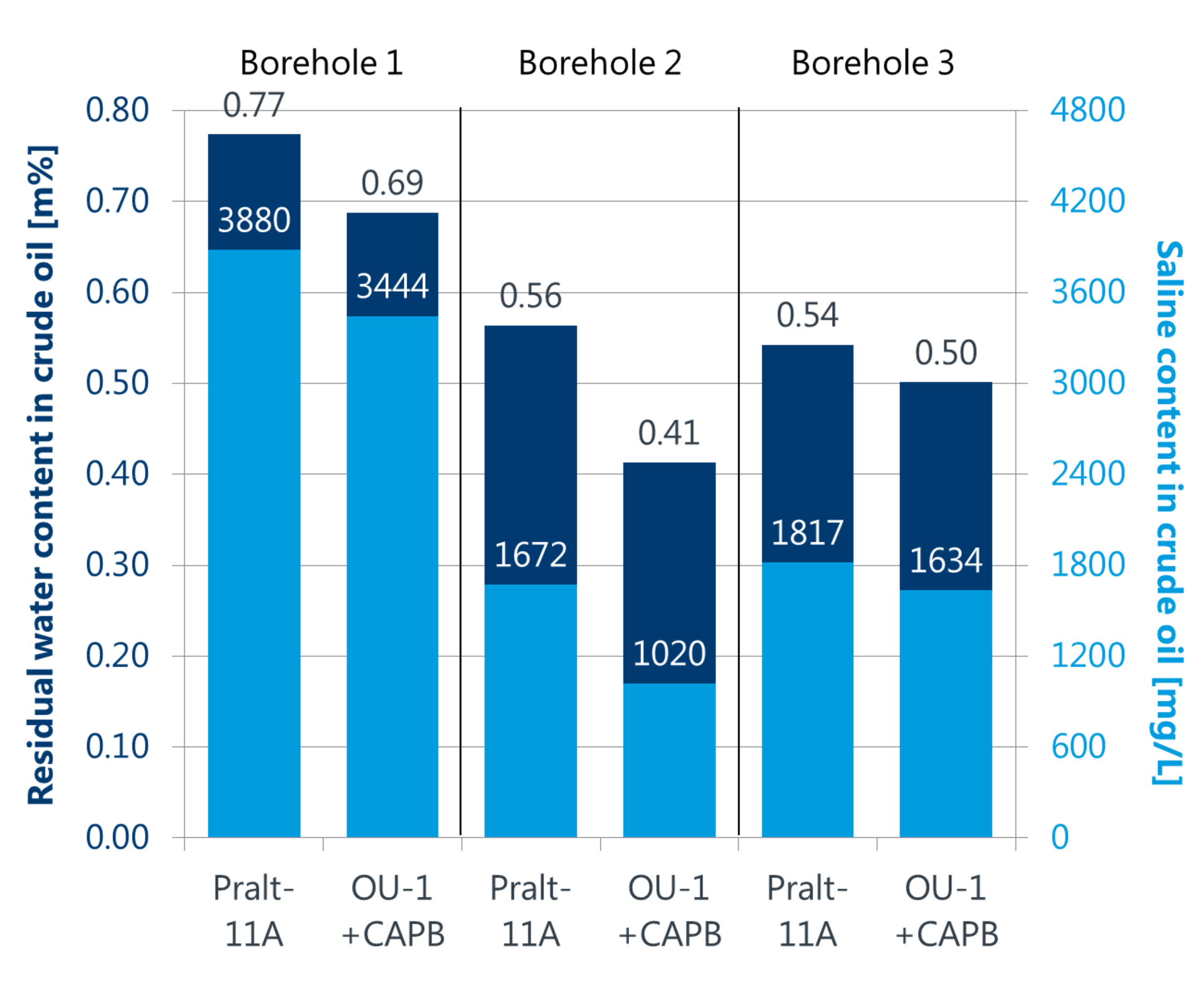
The system OU-1 + CAPB was used successfully directly in crude oil reservoirs. The pump pressure for transporting the oil was lowered and pressure variations reduced compared with an industrially used standard demulsifier (Pralt-11A) (Fig. 5).
Furthermore, the crude oil product obtained had a lower water and saline content (Fig. 6). In this way, it was therefore possible to optimize the overall crude oil production process with regard to demulsifier use from a simple model experiment.
Summary
The effect of different demulsifier systems on the structural mechanical strength of the asphaltene/toluene-water interface was investigated using the oscillating drop method. The storage modulus determined from these interfacial rheological experiments enables a conclusion to be drawn relating to the stability of (w/o) emulsions and was used here to determine the optimum demulsifier system. The mixed system OU-1 + CAPB, which resulted in the greatest reduction in storage modulus, was chosen to test the effects on pump pressure and water and saline content in practice. The selected demulsifier system led to lower pump pressures and a reduced water and saline content in the crude oil compared with the reference demulsifier system which had previously been used in these crude oil drilling fields. The data presented here therefore show by way of example how simple and cost-effective interfacial rheological analyses are suitable for optimizing field processes in the crude oil industry.
Bibliography
- [1] R. Mingazov, Compositions for destroying water-in-oil emulsions based on oligourethanes and ionogenic surface active substances, doctorate thesis, Kazan National Research Technical University (Russia), 2012.
- [2] G. Loglio, R. Miller, A. Stortini, U. Tesei, N. Degli Innocenti, R. Cini, Non-equilibrium properties of fluid interfaces: aperiodic diffusion-controlled regime 2. Experiments, Colloid Surface A 1995, 95, 63.
- [3] F. Thomsen, KRÜSS Application Report AR 246: Dehnübungen für Tropfen, 2005.
- [4] D. M. Sztukowski, H. W. Yarranton, Rheology of Asphaltene-Toluene/Water Interfaces, Langmuir 2005, 21, 11651.